- Date
- 10th December 2019
- Categories
By Dr Nigel Monk, CREST
Our earlier blogpost, The Joy of Tests, introduced some rationale behind testing system elements such as cooking appliances in a scientific laboratory and mentioned the tests of an EPC bought in Kenya and brought to the UK. There is a full report on the MECS website but these are a few interesting outcomes from that work.
As a reminder, the CREST lab tests use water as a highly reproducible cooking load so the appliance can be characterised accurately. These insights allow comprehension of in-country cooking trials using ‘real’ meals and local foods. We use this graphic to structure the energy flow analysis.

The first graph below illustrates several points. The first is how the cooker’s simple mechanical controls allow a clear distinction between the phases of operation. ‘Pre-heating’ is simply bringing the whole – food, pan, cooker – up to a pre-set temperature and pressure above atmospheric conditions. ‘Cooking’ continues at this heightened temperature until the clockwork timer reaches zero. ‘Cooling’ after the power is removed follows an exponential decay made complex by the non-homogenous thermal mass. However, while the cooker remains connected to the power source, the ‘keep-warm’ function will arrest temperature decay to maintain the food at approximately 70°C. That’s good if you’re expecting it but bad if you’ve forgotten to disconnect after cooking, so a feature we’re not entirely happy about.

Overall, the graph above shows how temperatures cycle during operation, because control relies on full-power, on-off, simple averaging. The dwell time between pulses will extend during cooking as the appliance warms through. In fact, the energy absorbed by the cooking process itself, that is by the chemical changes in the food molecules, is relatively insignificant. All (almost!) the energy input during cooking is used simply to replenish energy radiated away from the outside of the cooker. Well, why don’t we insulate the cooker to slow the rate of loss? Indeed, early tests suggest we can easily reduce the rate by half but, as ever, there’s a balance that must be paid: Consider this, the air within the spaces inside the cooker has a mass of around 5 grams. Air is a fairly good insulator, particularly if you prevent it from moving around too much. If you replace that with 700g of the best insulator available, a form of aerogel, it would need to be more than 100 times better than air just to be as good, because it will absorb 100 times as much energy before retaining energy inside, offsetting any positive effect. Replace the air with 0g of vacuum though and now you have the best of both options – and another 1% inspiration needing bringing to fruition. I digress, that exciting piece of discovery will have to wait for another blog (and we can just put a wonder-bag over it anyway, instead).
The second graph below illustrates the balance that needs paying. It illustrates that the predominant factor controlling the quantity of parasitic thermal energy lost during cooking is the duration of the pre-heat phase. Obviously, that will be related to the quantity of food being heated, but it holds true in a remarkably linear relationship as the extended pre-heating tests confirm.
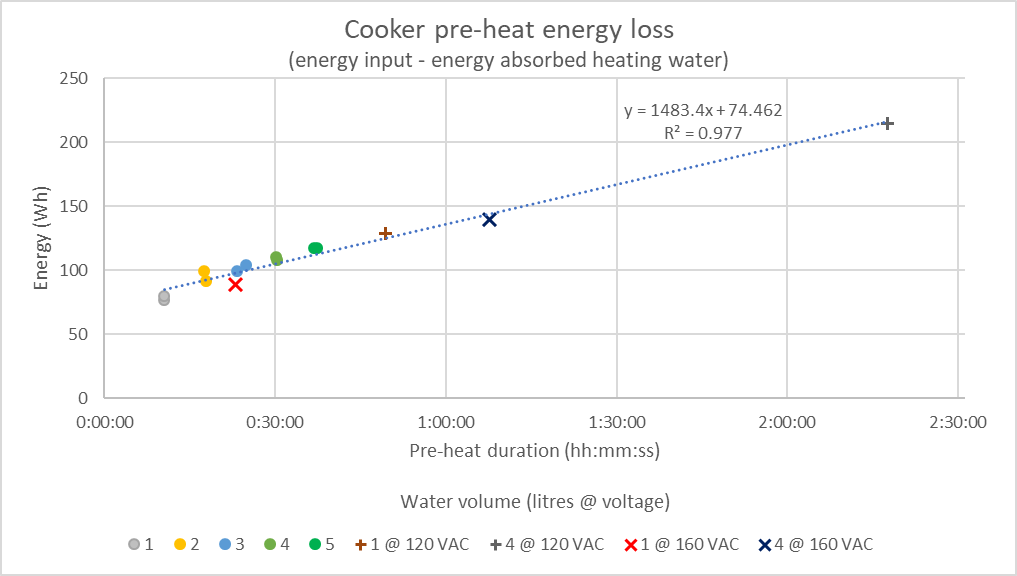
This is important because, should you wish to slow the pre-heating process, for example because you have designed a lower power appliance (to protect the battery capacity and life) and have thereby extended the pre-heat period, the associated energy losses will increase proportionately, and highly undesirably. Put two bags on it maybe?
More seriously, the fitted line above is characteristic of this cooker. Improving the cooker, by insulating the outside for example, should bring the line down (lower losses) and lower the slope. The characteristic can also be compared to results from other EPCs, as well as other appliance types. For other appliance types, we need to start to worry about appliance energy-conversion inefficiencies as well; did you know, a microwave oven wastes up to 50% of input energy in the process of converting it to microwaves? The wasted energy contributes to heating the converter (a magnetron) – that loud fan is nothing to do with clearing the steam from the oven, it is to cool the magnetron! I’ve digressed again.
The third graph adds some data to these EPC thoughts. At normal power, the pre-heating process is clearly more efficient when the cooker is fuller, despite the additional losses from the extended period, to over 80%. We tested the cooker at two lower voltages to represent half power and quarter power, equivalent to a 500W and 250W cooker respectively, to determine whether the energy input rate would overcome the energy loss rate so that the cooker achieved pressurisation. Otherwise, it would act like a rice cooker, and perhaps not even raise the food to cooking temperature. However, it did achieve pressurisation, albeit very slowly at the lower power. The graph shows how the associated increased losses reduce the proportion of energy input that is usefully absorbed into the water to as low as 45%.
